The research projects in our laboratory are focused on molecular imaging, molecular therapy, and nanotechnology. Molecular medicine is the future of 21st century patient management. Molecular imaging, “the visualization, characterization and measurement of biological processes at the molecular and cellular levels in humans and other living systems”, can play pivotal roles in disease diagnosis, treatment efficacy assessment, drug discovery, and the understanding of fundamental biology. In addition, molecular imaging is also an extremely powerful tool in multiple disciplines of biomedical research (e.g. stem cell research, RNA interference, nanomedicine, gene/cancer therapy, etc.). The molecular imaging techniques routinely used in our research include positron emission tomography (PET), bioluminescence, fluorescence, magnetic resonance imaging (MRI), among others.
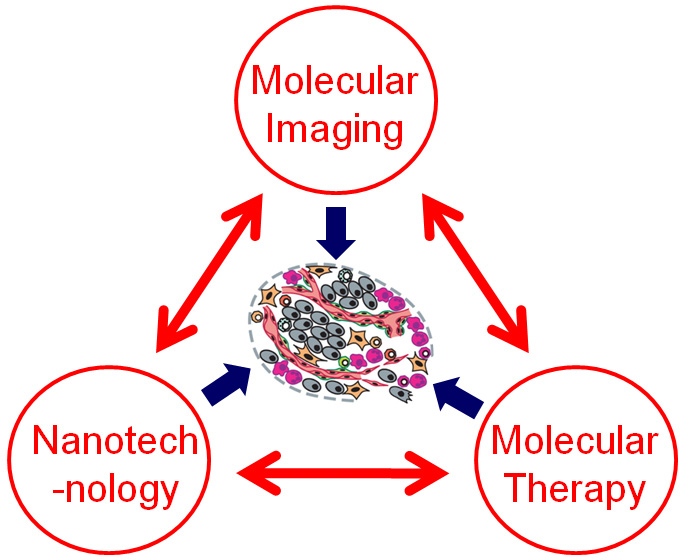
A. Development of novel PET tracers
18F-FDG PET is routinely used in the clinic for cancer patient management. The development of novel PET tracers has excellent translational potential, where they can be used for disease diagnosis, patient stratification, monitoring the therapeutic efficacy, among others, thereby ushering in a new era of “personalized medicine”. UW - Madison has a GE PETtrace (16.5 MeV) and a CTI RDS 112 (11 MeV) cyclotron with > 2500 ft2 of radiochemistry laboratory space. In 2006, the first Inveon microPET/microCT hybrid scanner from Siemens was installed at UW - Madison. This state-of-the-art scanner provides unsurpassed PET sensitivity (> 10%), resolution (1.2 mm), axial field of view (13 cm), 15 micron spatial CT resolution, and real time image reconstruction. Over the last several decades, our cyclotron team has produced more than 100 PET isotopes. The isotopes that are involved in our research may include 11C (t1/2: 20.4 min), 18F (t1/2: 109.7 min), 64Cu (t1/2: 12.7 h), 68Ga (t1/2: 67.7 min), 86Y (t1/2: 14.7 h), 89Zr (t1/2: 3.3 d), 124I (t1/2: 4.2 d), arsenic isotopes, among many others.
1. Non-Peptidic PET tracers
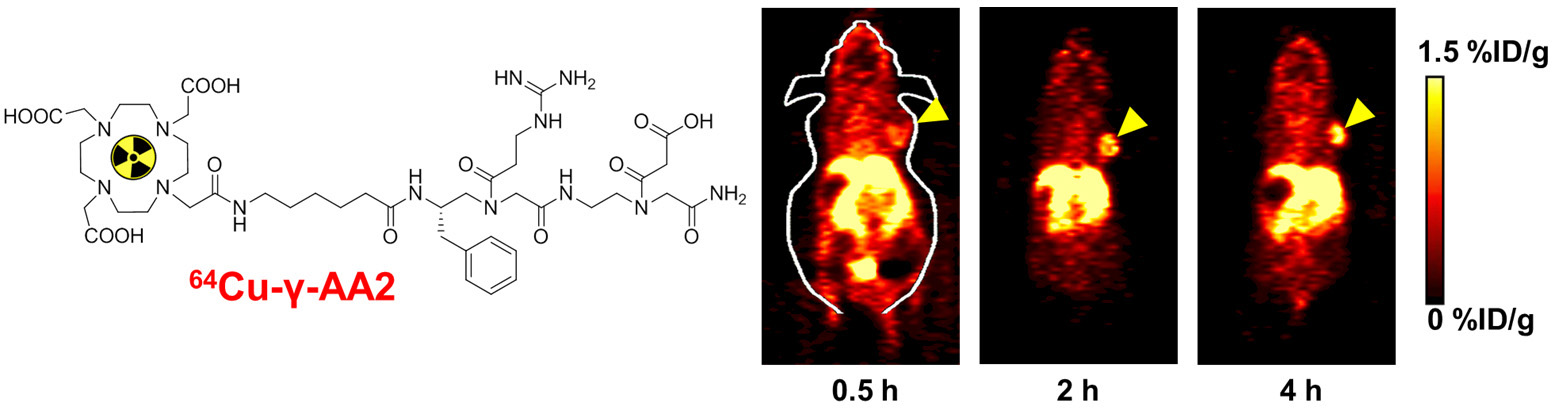
2. Peptide-based PET tracers
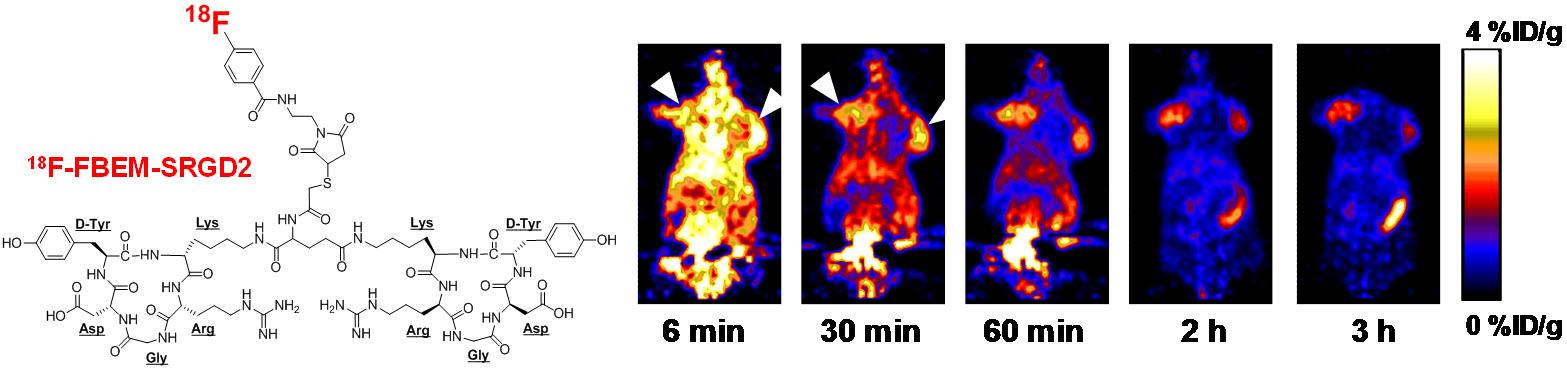
3. Protein-based PET tracers
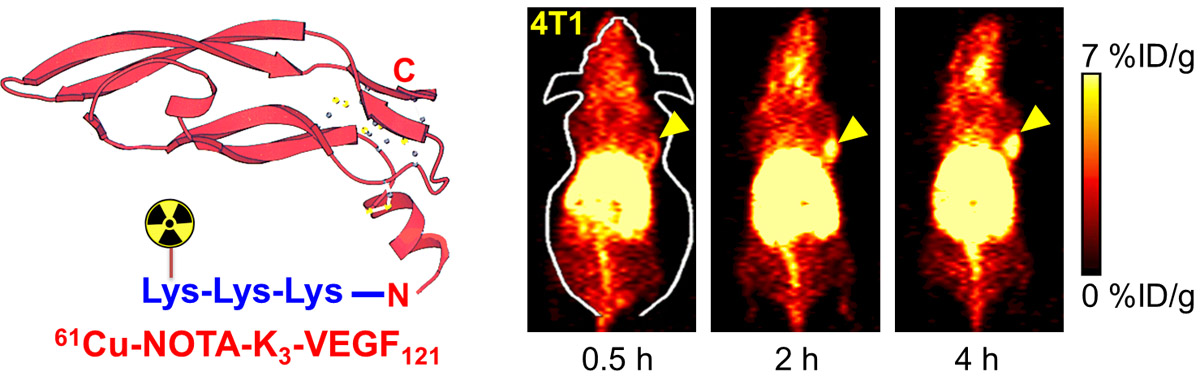
4. Antibody-based PET tracers
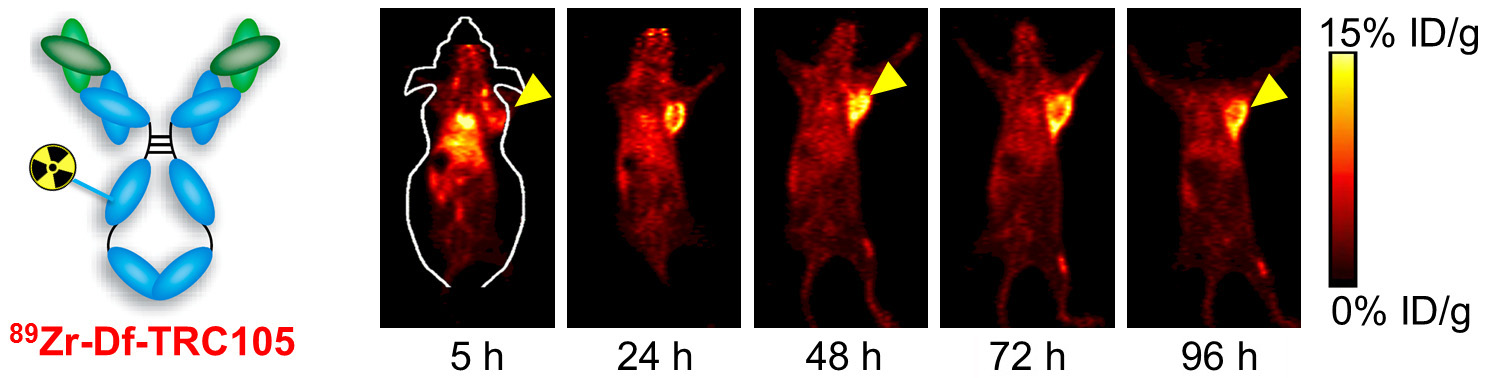
5. Antibody fragment-based PET tracers
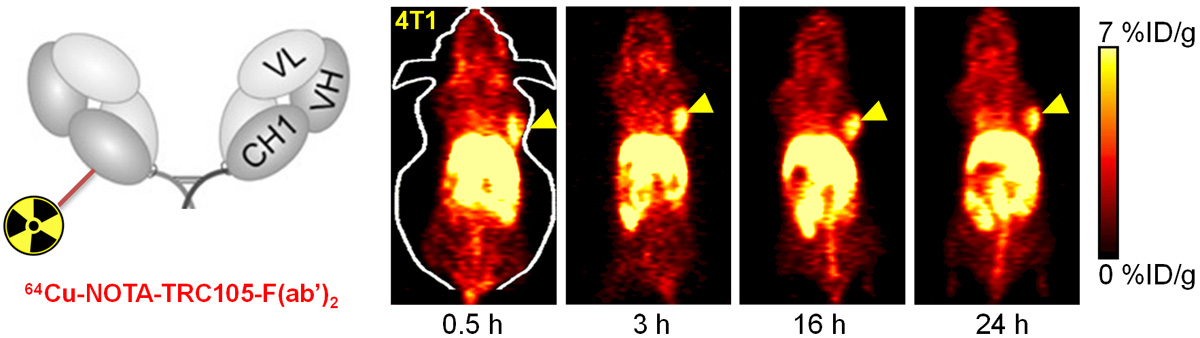
B. Nanotechnology
Nanotechnology is playing an increasingly important role in molecular imaging and therapy. The use of a molecularly targeted nanoplatform affords many advantages over conventional approaches. First, hundreds, thousands, or even more imaging labels or a combination of labels for different imaging modalities can be attached to a single nanoparticle, which can lead to dramatic signal amplification. Second, multiple, potentially different, targeting ligands on the nanoparticle can provide enhanced binding affinity and specificity. Third, the ability to integrate a means to bypass biological barriers can enable enhanced targeting efficacy. Ultimately, the combination of different targeting ligands, imaging labels, therapeutic drugs, and many other agents may allow for effective and controlled delivery of therapeutic agents in patients, which can be non-invasively monitored in real time. Nanotechnology can shed new light on molecular diagnostics and therapy. We have worked with a wide variety of nanomaterials, exploring their biomedical applications.
1. Nano-graphene
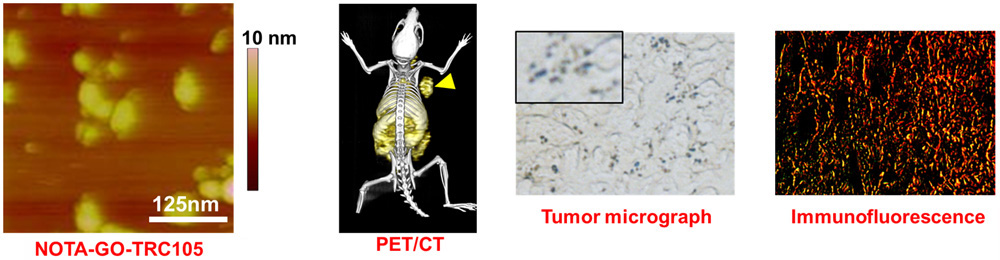
2. Zinc oxide nanowires
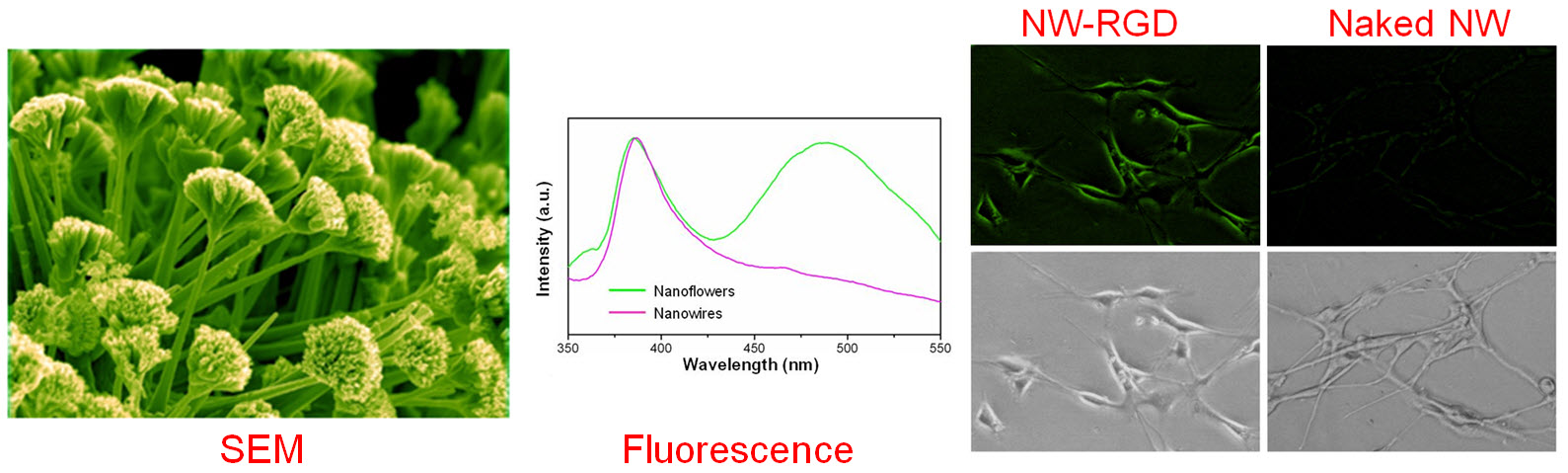
3. Mesoporous silica nanoparticles
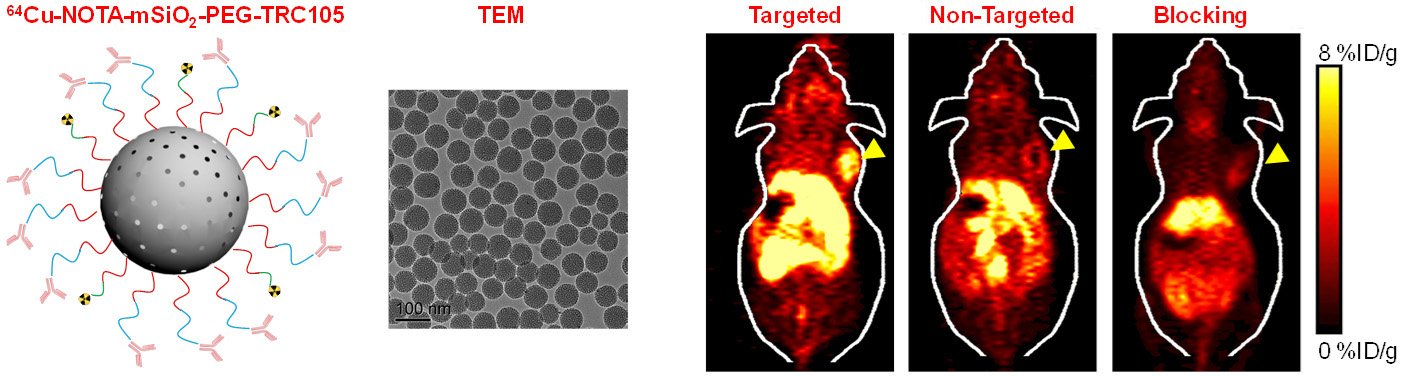
4. Hollow mesoporous silica nanoparticles
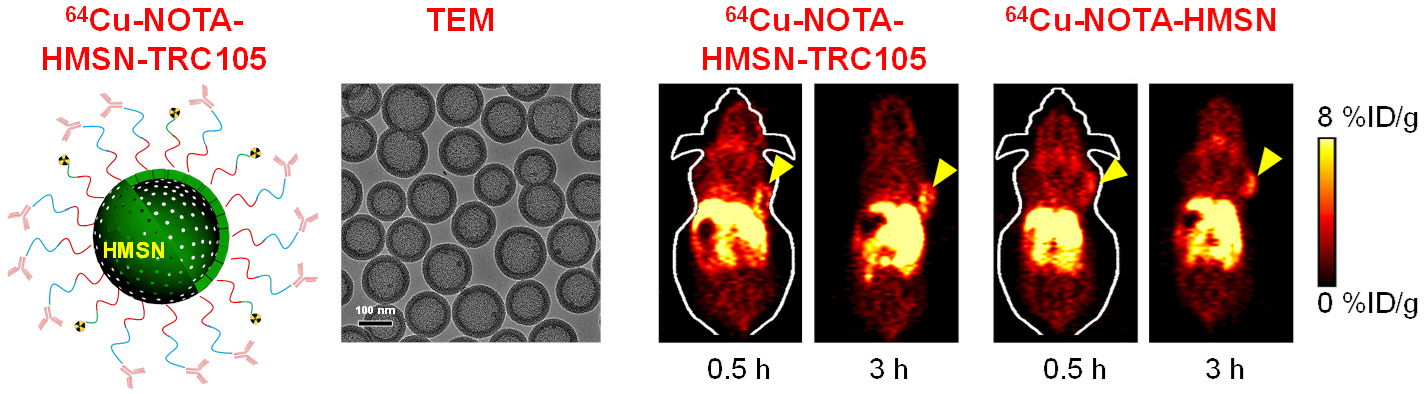
5. Single-walled carbon nanotubes (SWNTs)
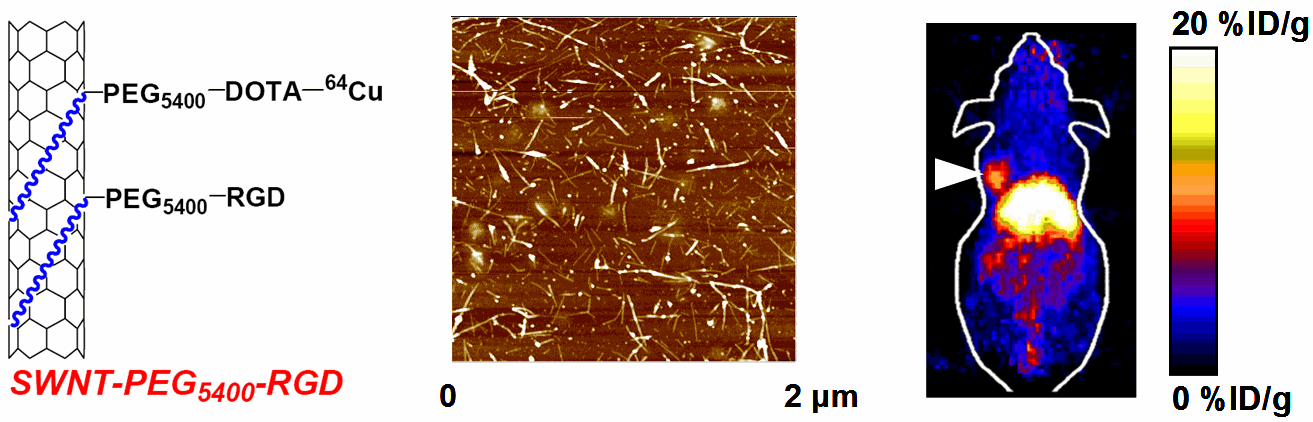
6. Superparamagnetic Iron oxide (SPIO) nanoparticles
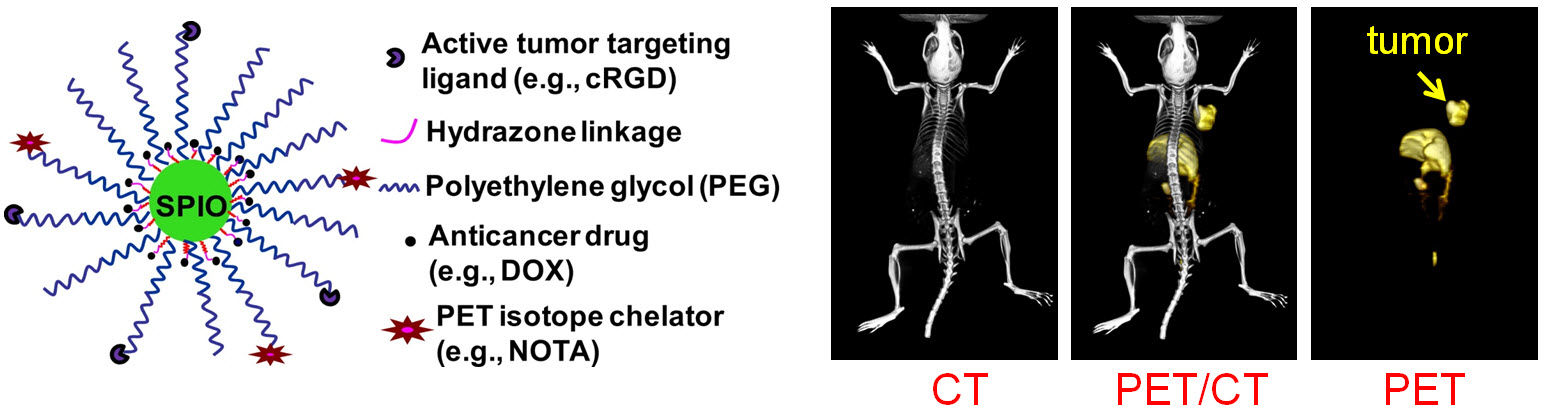
7. Unimolecular micelles
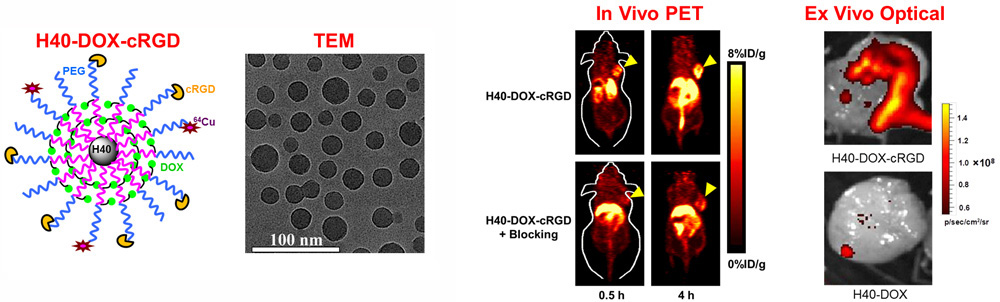
8. Gold nanorods
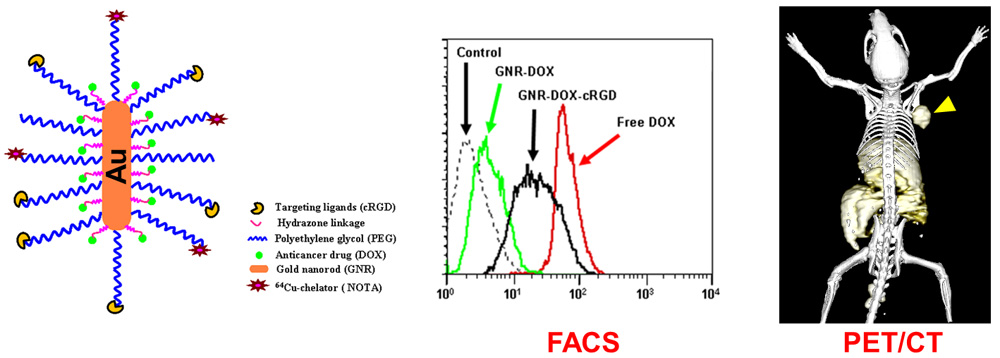
9. Quantum dots
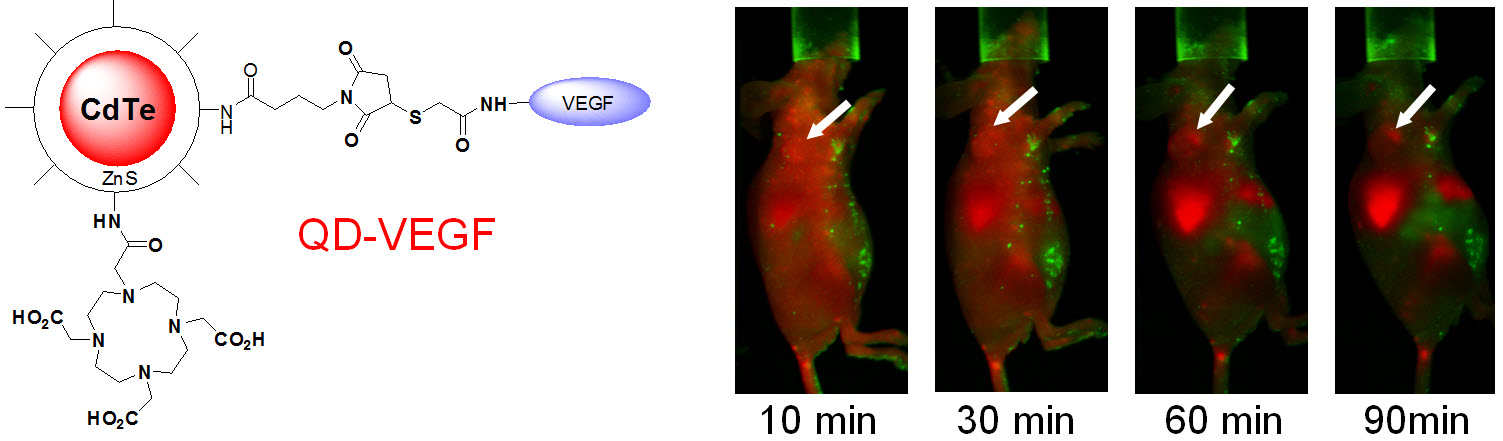
C. Develop of near-infrared fluorescence (NIRF) imaging agents
Optical imaging, which includes both bioluminescence and fluorescence, is a relatively low-cost method suitable primarily for small animal studies. The major types of contrast agents used for fluorescence imaging include organic dyes, fluorescent proteins, and quantum dots. For in vivo applications, imaging in the near-infrared (NIR; 700 - 900 nm) region, where the absorbance spectra for all biomolecules reach minima thus providing a clear optical window, can provide better opportunities for visualizing biological events in both small animal models and various clinical scenarios.
In 2009, an IVIS Spectrum system (Caliper Life Sciences) was installed at UW - Madison, in which both bioluminescence and fluorescence imaging can be achieved across the visible to NIR region. This system also offers single-view 3D tomography for both fluorescent and bioluminescent reporters, analyzed in the anatomical context of a digital mouse atlas.
1. Fluorescent dye-based agents
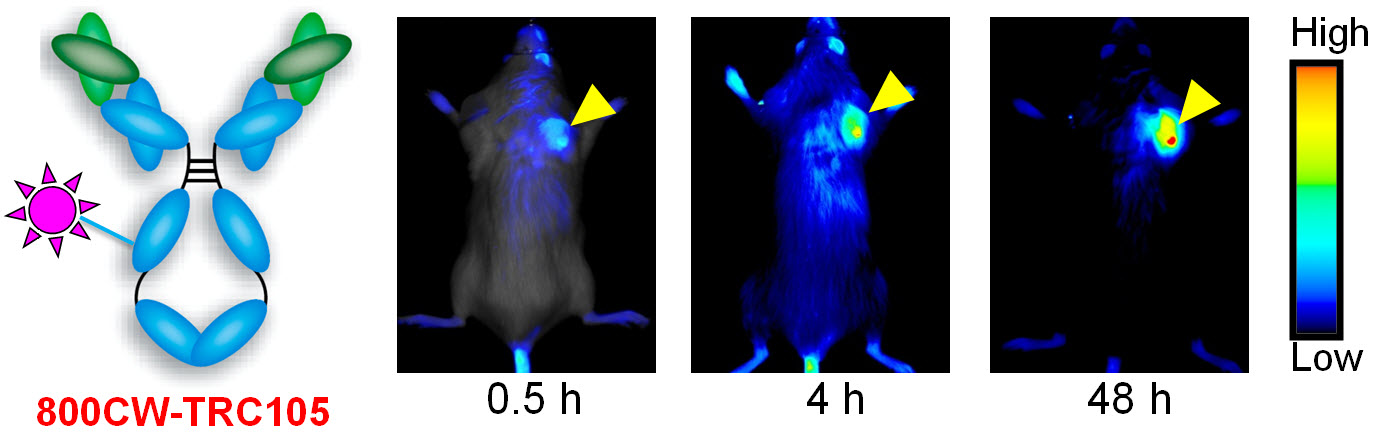
2. Quantum dot-based agents
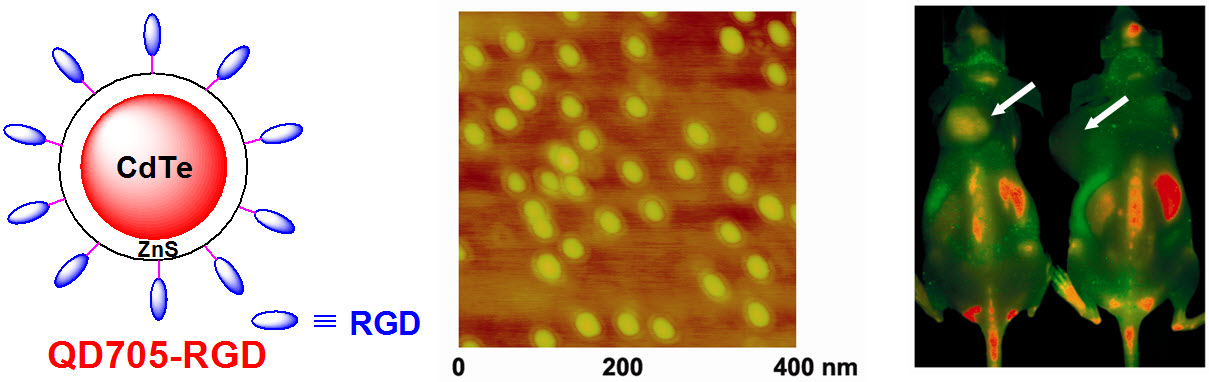
D. Development of multimodality imaging agents
Among all molecular imaging modalities, no single modality is perfect and sufficient to obtain all the necessary information for a particular question. For example, it is difficult to accurately quantify fluorescence signal in living subjects based on fluorescence imaging alone, particularly in deep tissues; MRI has high resolution and exquisite soft tissue contrast yet it suffers from very low sensitivity; Radionuclide-based imaging techniques are very sensitive and highly quantitative but they have relatively poor spatial resolution. Combination of certain molecular imaging modalities can offer synergistic advantages over any single modality alone. There are many different combinations depending on the goal of a particular study.
1. PET/MRI agents
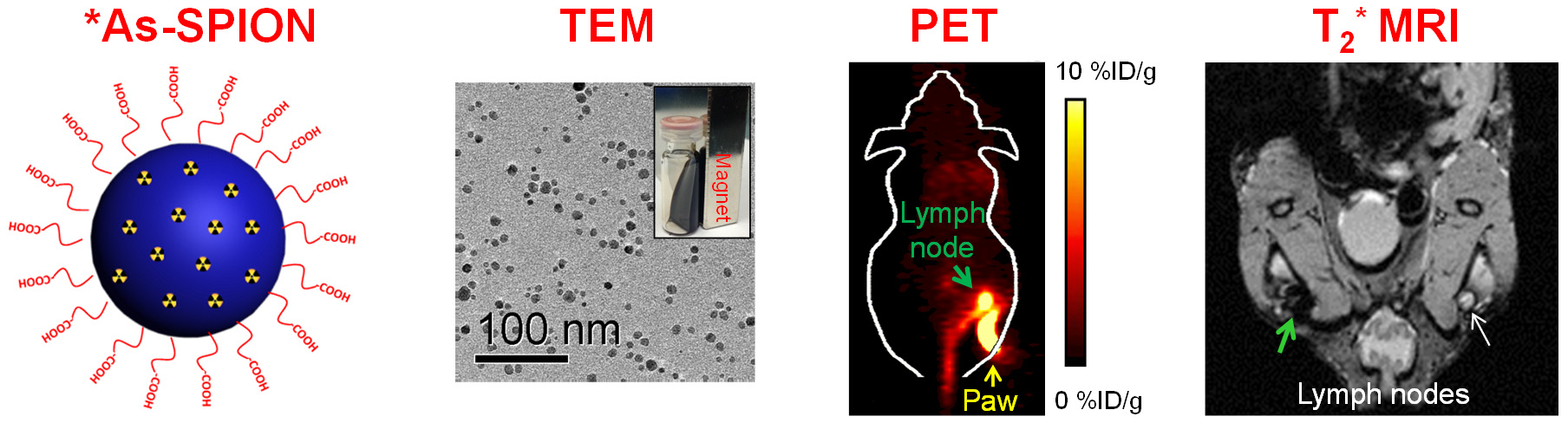
2. Nanoparticle-based PET/optical agents
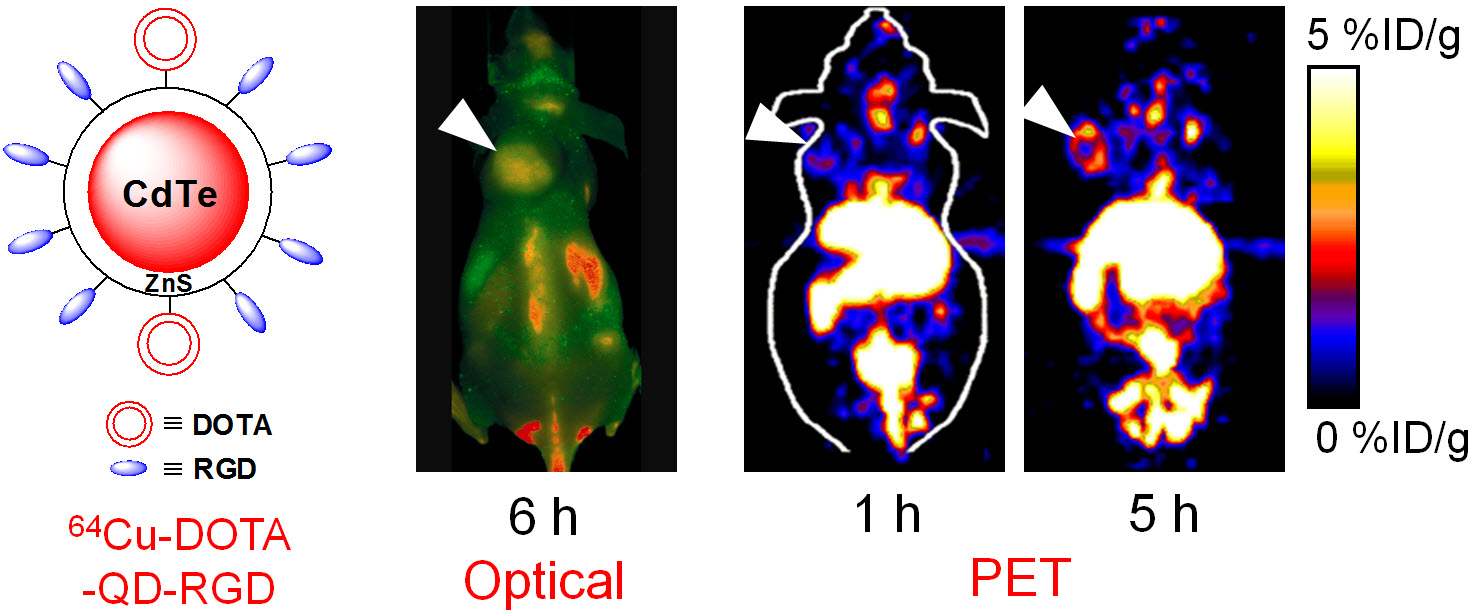
3. Non-nanoparticle-based PET/optical agents
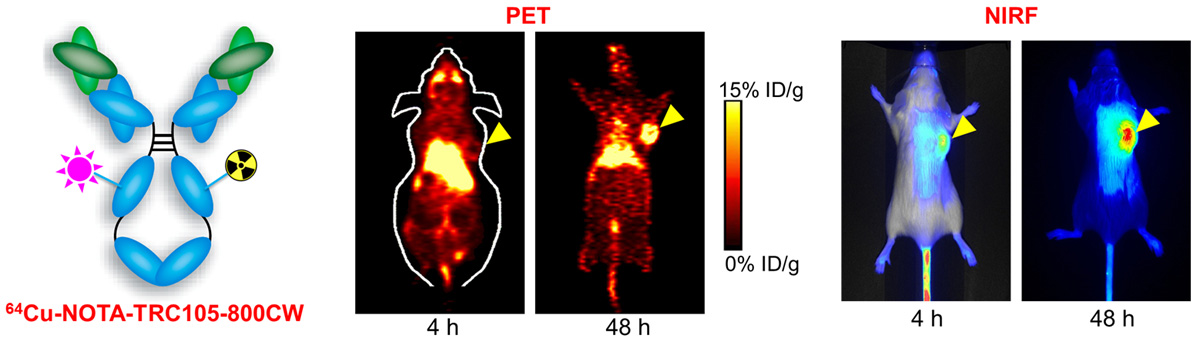
E. Theranostics
Theranostics can be used to describe the proposed process of diagnostic therapy for individual patients, i.e. to test them for possible reaction to taking a new medication and to tailor a treatment for them based on the test results. For example, non-invasive molecular imaging can be used to select the right patients for the right drug; an PET isotope-labeled antibody can be used to evaluate its pharmacokinetics, dosimetry, tumor targeting efficacy, etc, before a large therapeutic dose of (high energy β-emitter-labeled) antibody is administered; imaging can be used to monitor/guide the delivery of drugs; etc.
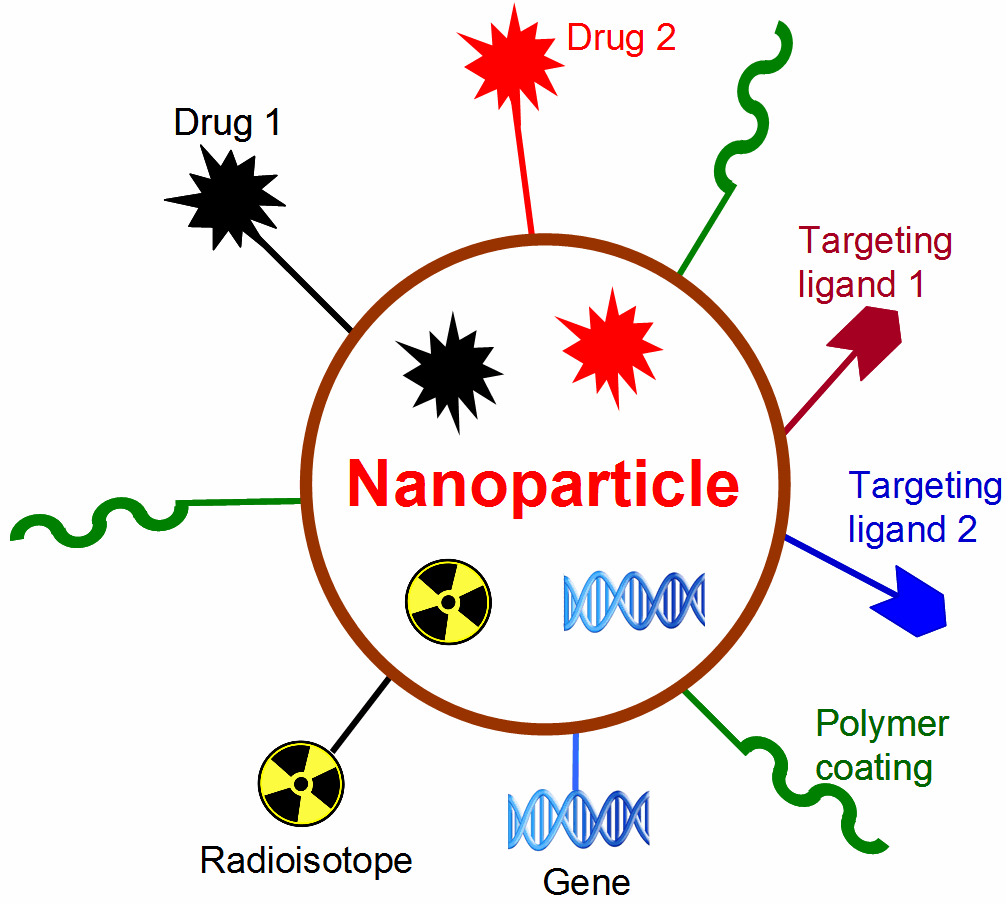
F. Molecular therapy of cancer
Molecular imaging can significantly speed up the drug development process while reducing the cost at the same time. Molecular imaging can be used to monitor the drug in blood, normal, and tumor tissues, as well as to measure the changes at the molecular level in vivo, thereby allowing for early assessment of the therapeutic efficacy. Molecular imaging can give whole body readout in an intact system, help to decrease the workload and speed up the drug development process, provide more statistically relevant results because longitudinal studies can be carried out in the same animals, aid in lesion detection and patient stratification, and help in individualized anti-cancer treatment monitoring and dose optimization.
1. Fusion proteins
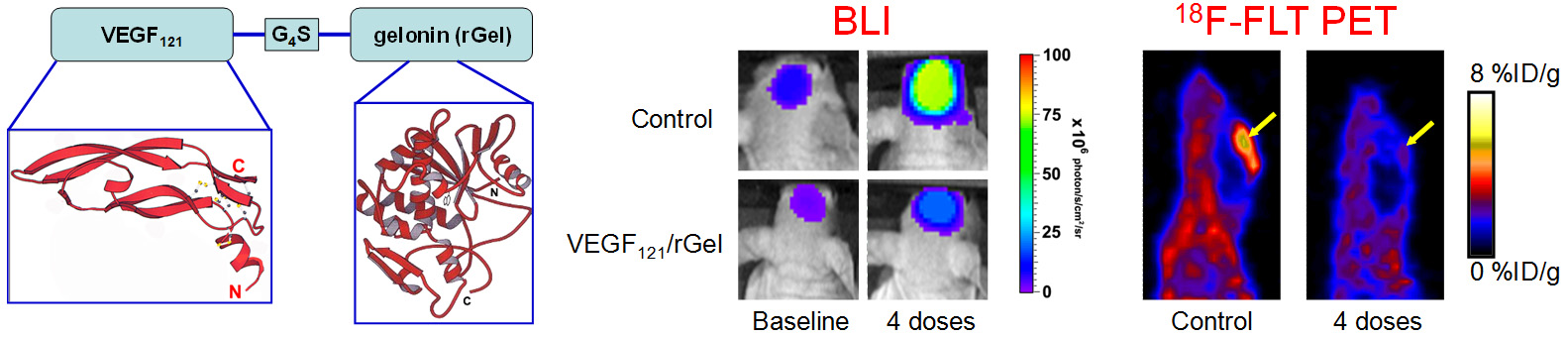
2. Peptide-drug conjugates
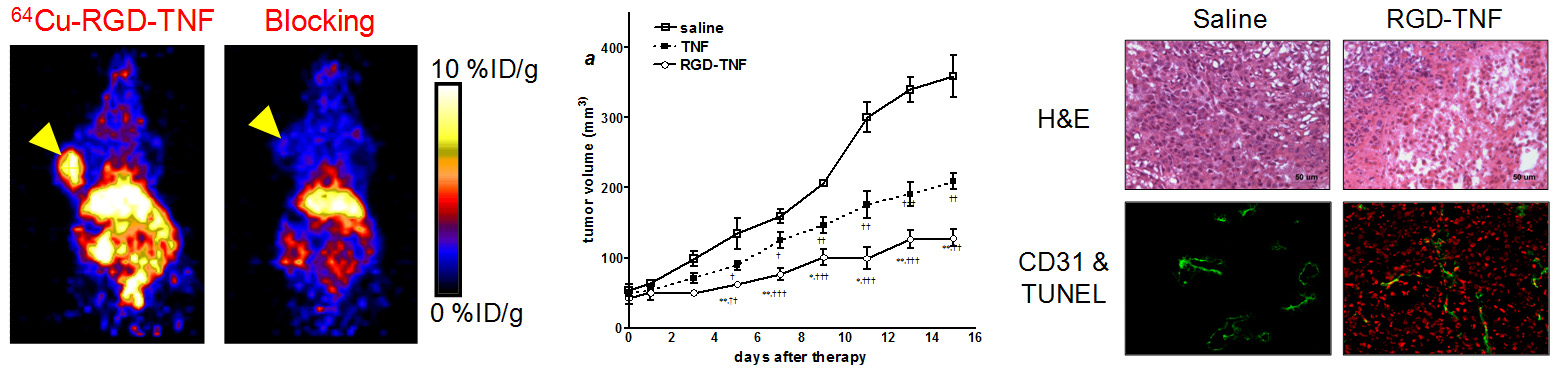
3. Radioimmunotherapy agents
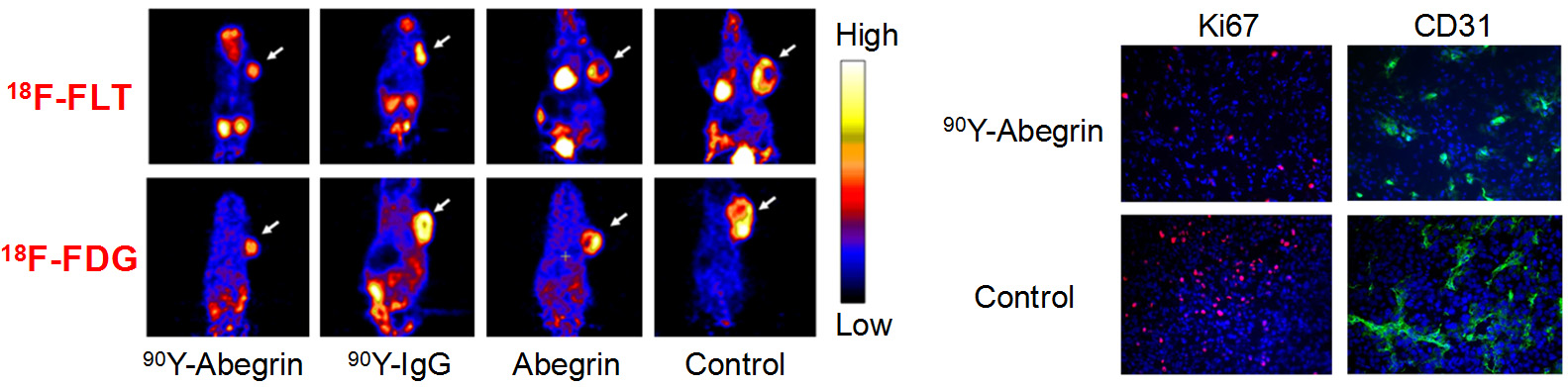
4. Small molecule drugs
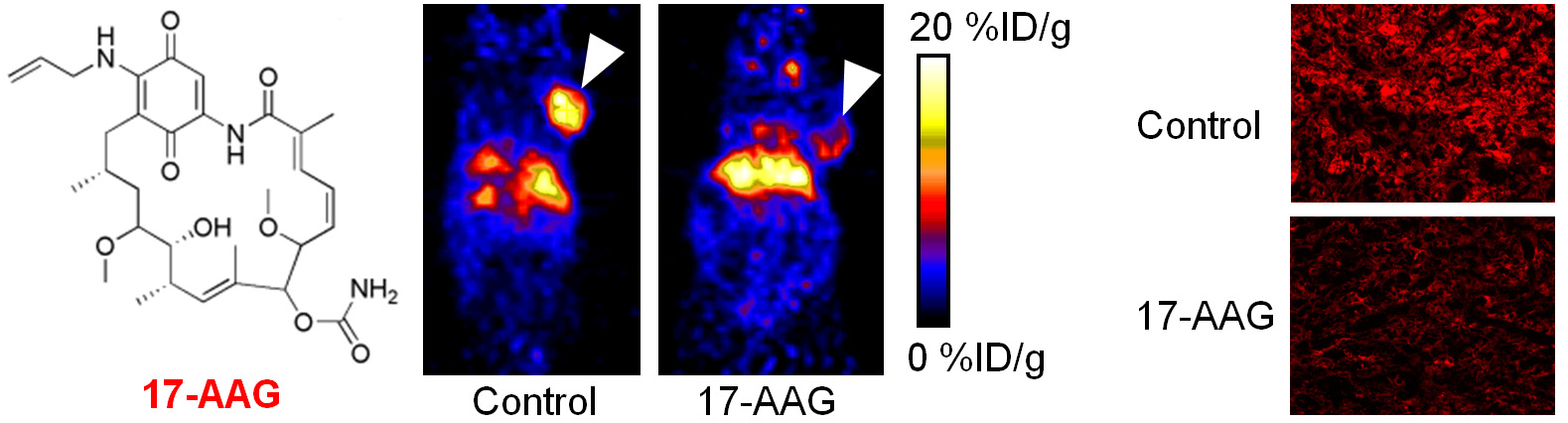
G. Development of reporter gene systems
Among the many molecular imaging techniques, reporter gene imaging has been a dynamic area of research. A wide variety of reporter genes and reporter probes have been developed, with a few of them already entering clinical investigation. The most widely-used reporter genes include those for optical (both fluorescence and bioluminescence) and radionuclide-based imaging. Many other reporter genes have also been reported for magnetic resonance imaging. One area of our research is to develop a reporter gene/reporter probe system for multimodality imaging in living subjects.

[ return to top ]
|